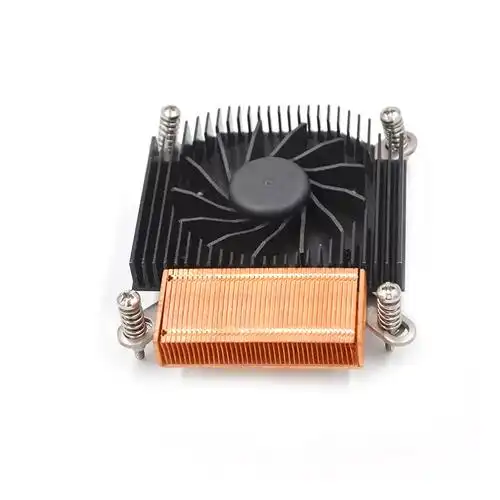
Modern processors generate staggering amounts of energy during operation. Without a critical cooling component, even the fastest systems would overheat in minutes. This unsung hero quietly battles thermal chaos through physics-driven design.
Metal devices with fins or ridges attach directly to processors, pulling energy away through conduction. Materials like aluminum and copper excel here due to their atomic structure, which enables rapid heat transfer. Engineers optimize shapes to maximize surface area, allowing air or liquid cooling systems to finish the job.
Effective thermal management depends on two factors: material science and mechanical engineering. High conductivity metals form the base, while precisely arranged channels accelerate airflow. Advanced designs even use vapor chambers to spread energy across larger areas before dissipation.
Matching these components to a computer’s power demands prevents throttling and extends hardware life. Gamers and professionals alike benefit from tailored solutions that balance noise levels with cooling capacity. As processors grow more powerful, innovative thermal designs become non-negotiable for peak performance.
Introduction to CPU Heat Sinks
Computing power relies on precision thermal management to prevent catastrophic failures. Cooling systems evolved from basic metal plates to engineered solutions combining passive and active elements. This progression reflects growing demands for energy-efficient heat dissipation in compact spaces.
Overview of CPU Cooling
Passive thermal management forms the foundation of processor protection. Metals with high thermal conductivity absorb energy from silicon chips, while extended surfaces accelerate heat release. Airflow patterns across ridges and fins turn ambient movement into cooling power.
Active systems enhance this process with rotating fans. These components push air through tight channels, boosting convection rates. Combined approaches balance noise reduction with rapid temperature control, adapting to workloads from office tasks to 4K rendering.
Importance of Effective Heat Dissipation
Excessive temperatures degrade silicon integrity and throttle clock speeds. Proper cooling maintains stable frequencies during extended workloads. Material selection directly impacts longevity—copper bases outperform aluminum in high-wattage scenarios due to superior electron mobility.
Optimized surface area designs multiply cooling potential without increasing footprint. Thermal interface materials fill microscopic gaps between components, ensuring efficient energy transfer. Systems lacking these considerations risk premature hardware failure during peak demands.
Principles of Heat Transfer and Cooling Methods
Heat movement in electronics follows three core mechanisms. Each plays a role in managing energy flow from hot components to cooler environments. Designers leverage these principles to create efficient thermal solutions.
Conduction, Convection, and Radiation
Conduction moves energy through direct contact. Metals like copper excel here due to atomic structures that allow rapid electron movement. This process forms the first stage of cooling, transferring heat from processors to heat sinks.
Convection occurs when air or liquid carries energy away. Fans accelerate this process by forcing airflow across finned surfaces. The temperature difference between components and ambient air determines cooling speed.
Radiation emits infrared waves from hot surfaces. While less impactful in computers, dark-colored materials enhance this effect slightly. Most systems prioritize conduction and convection for rapid results.
Transfer Mode | Mechanism | Key Influencers |
---|---|---|
Conduction | Direct contact | Material conductivity, contact quality |
Convection | Fluid movement | Airflow speed, surface area |
Radiation | Electromagnetic waves | Surface emissivity, temperature |
Understanding Thermal Resistance
This measurement quantifies how easily heat flows through materials. Lower resistance means better thermal conductivity. Poor contact between components creates bottlenecks, reducing cooling efficiency.
Designers combat resistance by using premium thermal interface compounds and optimizing fin density. High-performance systems often combine copper bases with aluminum fins to balance cost and capability.
Materials and Design Considerations
Engineers face critical decisions when selecting components for thermal management systems. Material properties and structural geometry directly determine how efficiently energy moves from processors to the environment.
Aluminum vs. Copper: Pros and Cons
Copper dominates high-performance applications with 60% greater thermal conductivity than aluminum. Its atomic structure enables rapid energy transfer from hot spots to cooling fins. However, copper’s density triples aluminum’s weight, complicating mounting in portable systems.
Aluminum offers cost-effective solutions for moderate workloads. Its lightweight nature suits compact devices, though thicker bases compensate for lower conductivity. Oxidation resistance gives aluminum durability advantages in humid environments.
Material | Conductivity (W/mK) | Density (g/cm³) | Cost Index |
---|---|---|---|
Copper | 401 | 8.96 | 3.2 |
Aluminum | 237 | 2.70 | 1.0 |
Composite and Advanced Materials
Hybrid designs combine copper bases with aluminum fins to balance cost and performance. Emerging composites like graphene-enhanced alloys achieve 450 W/mK conductivity while reducing weight. Vapor chamber integration spreads energy across entire surfaces before fin dissipation.
Ceramic-metal matrices now enable custom conductivity patterns. These innovations allow targeted cooling for multi-chip modules while maintaining structural integrity under thermal stress. Material advances continue pushing the boundaries of compact thermal solutions.
What is a cpu heat sink: Key Components and Features
Advanced thermal solutions combine multiple engineered elements to manage energy flow. Each component plays a distinct role in moving heat from silicon chips to the surrounding environment. Precision engineering ensures these parts work together seamlessly under varying workloads.
Fins, Heat Pipes, and Fans Explained
Extended fins multiply surface area for faster heat dissipation. Thin aluminum ridges in budget coolers contrast with copper-nickel arrays in premium units. Heat pipes containing coolant vapor transport energy from base to fins 40% faster than solid metal alone.
Rotating fans accelerate airflow across fin stacks. High-static-pressure models excel in tight spaces, while larger diameter designs move more air quietly. Gaming rigs often pair copper heat pipes with PWM-controlled fans for dynamic thermal management.
Thermal Interface Materials and Contact Efficiency
Interface compounds bridge microscopic gaps between processors and cooling bases. Silver-based pastes outperform silicone pads by 15°C in stress tests. Liquid metal solutions push boundaries but require careful application.
Proper mounting pressure ensures full contact across the heat spreader. Premium coolers use spring-loaded screws and backplates to maintain consistent force. Even the best materials fail if air pockets form between surfaces.
Interface Type | Conductivity (W/mK) | Application |
---|---|---|
Silicone Paste | 0.8-3.5 | Consumer PCs |
Carbon-Based | 5-12 | Overclocking |
Liquid Metal | 73+ | Extreme Systems |
Heat Sink Attachment Methods and Installation Practices
Securing cooling components correctly determines their ability to manage energy flow. Proper installation techniques prevent air gaps and maintain consistent pressure across surfaces. Even premium materials underperform if attachment methods compromise contact quality.
Mechanical Mounting Techniques
Spring-loaded brackets and screw-based systems dominate modern builds. These mechanisms apply uniform force as metals expand and contract during temperature shifts. Vibration-resistant clips lock components in place during transportation or intense workloads.
Backplates distribute mounting pressure evenly across the processor. Copper bases paired with steel fasteners handle repeated thermal cycling without warping. Over-tightening remains a common error—torque specifications ensure optimal clamping force without damaging silicon.
Thermal Adhesives and Compounds
Interface materials bridge microscopic imperfections between surfaces. High-viscosity pastes stay in place during installation, while pre-applied pads simplify DIY builds. Silver-based compounds outperform standard options by 20% in stress tests.
Pea-sized application methods prevent overflow onto sensitive circuitry. Curing times vary—some materials require 24 hours before handling thermal loads. Reusing old adhesive layers creates insulating barriers that throttle cooling efficiency.
Industry guidelines emphasize surface preparation. Isopropyl alcohol removes oxidation before applying fresh thermal interface materials. Periodic reseating maintains peak performance as components age and settle.
Engineering Insights and Real-World Applications
Thermal solutions bridge theoretical physics with practical engineering demands across industries. Design innovations emerge through rigorous testing cycles simulating extreme workloads, pushing materials and geometries to their limits.
Cooling in Consumer and Gaming PCs
High-performance gaming rigs demand compact solutions with rapid heat transfer. Copper bases paired with nickel-plated fins dominate premium builds, handling 250W thermal loads during overclocking. PWM-controlled fans adjust speeds based on real-time temperature sensors.
Liquid-cooled systems now integrate hybrid designs. Vapor chambers spread energy across entire surfaces before aluminum radiators dissipate it. These setups maintain CPU clock speeds under 4K rendering workloads without audible noise spikes.
Industrial Uses and High-Power Systems
Manufacturing equipment requires robust cooling for continuous operation. Forged copper heat sinks with pressurized mounting systems handle 500W+ loads. Active airflow management combats dust accumulation in harsh environments.
Data centers employ phase-change materials in server racks. These solutions absorb sudden thermal spikes during peak computations. Thermal interface compounds here withstand 10,000+ thermal cycles without degradation.
Application | Key Features | Materials | Thermal Capacity |
---|---|---|---|
Gaming PCs | Dynamic fan control | Copper/aluminum hybrids | 150-300W |
Industrial Systems | Dust-resistant fins | Solid copper bases | 400-800W |
Leading manufacturers now test prototypes in climate-controlled chambers for 1,000+ hours. This ensures stable performance across temperature extremes from -40°F to 185°F. Such validation separates consumer-grade solutions from industrial-grade reliability.
Innovations in Cooling Efficiency and Design
Cutting-edge thermal solutions now leverage computational modeling to push cooling boundaries. Engineers combine material science breakthroughs with airflow dynamics, achieving unprecedented efficiency in compact spaces.
Surface Area Optimization and Fin Efficiency
Staggered fin arrangements increase turbulence without adding bulk. These patterns disrupt laminar airflow, boosting convective heat transfer by 18% in lab tests. Fractal-inspired designs now pack 40% more surface area into standard footprints.
Thin-gauge aluminum fins with copper cores demonstrate hybrid advantages. This approach balances lightweight construction with rapid energy dispersion. Advanced stamping techniques create micro-channels that accelerate airflow between ridges.
Design Feature | Surface Gain | Noise Reduction |
---|---|---|
Traditional Fins | Base 100% | 0 dB |
Staggered Array | +27% | -2.1 dB |
Fractal Geometry | +41% | -3.8 dB |
Design Innovations for Low-Noise Performance
Fluid dynamic bearings now enable near-silent fan operation below 15 dBA. These systems maintain 2200 RPM speeds while reducing mechanical wear. Strategic blade spacing eliminates harmonic vibrations that cause audible hums.
Case studies reveal impressive results. A popular gaming cooler reduced noise by 40% using angled intake shrouds and rubber isolators. These modifications maintained 95% cooling capacity during 4K rendering benchmarks.
Phase-change materials embedded in radiator blocks absorb thermal spikes quietly. This innovation reduces reliance on aggressive fan curves during sudden workload increases. Users enjoy stable performance without distracting sound profiles.
Conclusion
Maintaining stable operating temperatures remains foundational to computing reliability and speed. Efficient thermal management systems prevent performance throttling while extending hardware lifespan. These solutions combine physics principles with precision engineering to channel energy away from sensitive components.
Optimal designs balance material conductivity with intelligent airflow patterns. Copper bases and aluminum fins work synergistically, leveraging each metal’s atomic advantages. Advanced manufacturing now integrates vapor chambers and fractal geometries to maximize surface exposure.
Proper installation ensures full contact between processors and cooling components. Even premium heat sinks underperform without quality thermal interface materials. Regular maintenance prevents dust buildup that disrupts airflow across finned surfaces.
Future innovations will focus on hybrid materials and adaptive cooling architectures. As processors shrink while increasing power output, dissipation efficiency becomes critical. The evolution from basic metal plates to engineered systems demonstrates how thermal solutions shape computing progress.